Downloaded from rspb.royalsocietypublishing.org on July 20, 2010
4 A. E. Welchman et al. Reaction beats intention
(a) 800
750
700
<D
650
600
О
Q
550
500
450
(b) 30
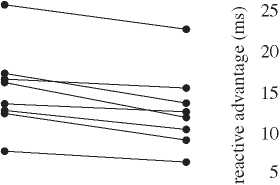

----------------------------- -5
initiate react
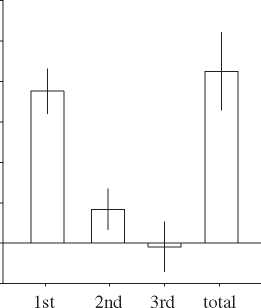
movement execution phase
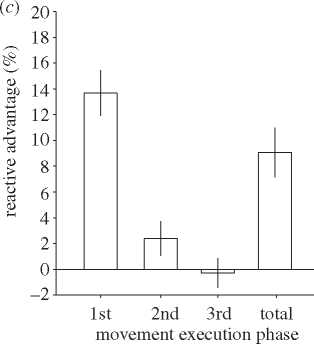
Figure 2. (a) Average movement execution times for each individual participant. Data show the harmonic mean execution time.
Points connected by lines indicate the data from a single individual. Our data analysis considered the difference between these
matched-pair responses. (b) The ‘reactive advantage’ (¼initiated movement execution time 2 reactive movement execution
time) for the three component phases of the movement sequence (1st: lift up from button 1, press down button 2; 2nd: lift
up button 2, press down button 3; 3rd: lift up button 3, press down button 1), and for the total execution time (lifting up
button 1 to pressing it down again having pressed button 2 and then 3). Data illustrate the between-subjects mean response.
Error bars show s.e.m. (c) The reactive advantage expressed as a percentage change in the mean execution time. Data illustrate
the between-subjects mean response with error bars showing s.e.m.
accuracy (Woodworth 1899). This in and of itself does
not constitute a trivial explanation for our findings, as
participants did not know a priori whether a reactive or
intentional movement would be made. Had the roles of
initiator and reactor been predetermined at the start of
a trial, data interpretation would be complicated. In par-
ticular, if participants knew that they would react on a
given trial, they could consciously elect to maximize
their chances of winning the duel by producing faster
and less accurate movements to compensate for the
time cost of reacting after their opponent. However, the
dynamic nature of the competition meant that this was
not possible: both participants prepared to initiate the
movement sequence. Thus, like a quickening of execution
times, a change in error rates suggests a dynamic compu-
tation of movement influenced by the type of movement
being produced. High-speed, low-accuracy movements
may constitute a characteristic of the neural systems
responsible for controlling reactive movements.
A potentially trivial difference between movements
executed under reactive and intentional conditions is that,
when making a reactive movement, participants had been
waiting longer to move. Under some circumstances
preparation time and movement speed are related
(Rosenbaum et al. 1987; Bullock & Grossberg 1988),
potentially suggesting a lurking variable between our two
movement classes. Our use of the covert random start
delay made this suggestion rather unlikely (the time from
the trial starting to the initiating participant’s movement
had a between-trial standard deviation of 775 ms in contrast
to typical reaction times of 200 ms). However, we tested
this idea by performing regressions of movement execution
time on the initiation time on a per subject basis, finding
no evidence for the relationship between preparation and
execution times under our experimental paradigm.
An additional concern might relate to the warning tone
that indicated participants had moved before the end of the
covert delay period. In particular, the tone might effectively
act as a ‘penalty’ that could change the movement strategy
so that participants were cautious, and thus slower, when
initiating the movement sequence. To test this idea, we
ran a control experiment on eight participants in which
the no intertrial delay was imposed. We found clear
evidence for a reactive advantage (t7 ¼ 8.426, p , 0.001)
when there was no warning tone, ruling out this concern
and suggesting that any penalizing effect of the warning
tone was not responsible for faster reactive movements.
These data were also useful in allowing us to test for
evidence of a speed-accuracy trade-off under our paradigm
(we could not do this for the main experimental data as
button press times were not recorded for error trials). In
particular, we considered the duration of the first move-
ment in the sequence of trials in which an error was
subsequently made (e.g. the participant missed button
3 or 1 during subsequent movements). We compared the
duration of these movements with those measured on
successful (non-error) trials, to test whether errors were
associated with faster movements. We found no evidence
for a difference between error and non-error movement
times for either reactive (p ¼ 0.27) or intentional
(p ¼ 0.80) movement sequences.
Finally, our task requiring a movement sequence of
three distinct segments might be regarded as overly com-
plex, with the necessity of reversing the direction of travel
leading to uncertainty in hand position after the initial
movement has been made. In an additional control experi-
ment we asked participants to make a simple, single
segment movement (from button 3 to button 2) under
competition. Consistent with our previous findings,
we observed clear evidence for a reactive advantage (t7 ¼
3.852, p ¼ 0.006) for this simple ballistic movement.
(b) Experiment 2
To gain further insight into the reactive advantage, we
asked whether the effect might accrue from having the
opponent’s movement as a model for one’s own actions.
In particular, ventral premotor cortex is known to be
Proc. R. Soc. B