Journal of Vision (2007) 7(8):1, 1-12
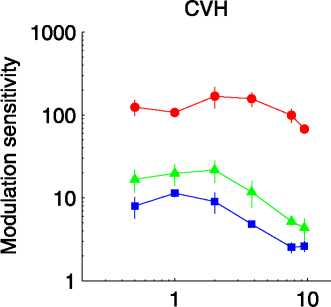
Figure 2. Sensitivity as a function of temporal frequency; details are as described in Figure 1.
Schofield, Ledgeway, & Hutchinson
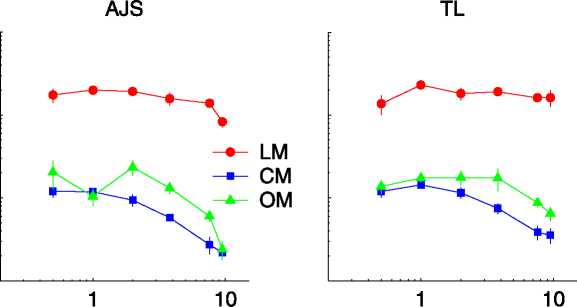
Temporal frequency (Hz)
2003). Further, Hutchinson and Ledgeway (2006) recorded
low-pass sensitivity for CM stimuli using an isotropic
dynamic.
Experiment 2
Sensitivity curves for direction discrimination versus
temporal frequency are shown in Figure 2. Sensitivity for
LM was quite flat, although it did decline at the highest
frequencies tested (again, the range of test frequencies
was not high enough to fully characterize the LM
response). Sensitivity curves for CM and OM were more
low pass, suggesting a low cutoff frequency for these cues
relative to that for LM. Crucially, CM and OM produced
very similar sensitivity curves in the presence of our 1-D
noise, with OM having perhaps a marginally higher cutoff
frequency. Thus, temporal acuity for OM is relatively
high, suggesting that OM is not a third-order cue as
defined by Lu and Sperling (2001).
Experiment 3: Motion aftereffect
Having first characterized the spatial- and temporal-
frequency responses to LM, OM, and CM in Experiments 1
and 2, we investigated suprathreshold interactions
between these cues using the dMAE paradigm.
Methods
Observers
Observers A.J.S., C.V.H., and P.D.J. participated in
Experiment 3.
Apparatus and stimuli
The apparatus and stimuli were similar to those used in
Experiments 1 and 2 with the following exceptions.
Adaptation stimuli were drifting patterns composed of
LM, CM, or OM (see Movies 1-3). The spatial and
temporal frequencies of the modulation were 0.5 c/deg
and 1 Hz, respectively, chosen based on the results of
Experiments 1 and 2 to ensure that each variety of motion
was readily visible and did not exceed its spatial and
temporal acuity limits. Adaptation stimuli were presented
at 10× the appropriate threshold value for A.J.S. and
C.V.H. and 8.5 × threshold for P.D.J. (it was impossible to
generate adaptation stimuli at 10× P.D.J.’s CM threshold
at 0.5 c/deg). Threshold estimates were taken from
Experiment 1.
The test stimuli (Movies 4-6) were directionally
ambiguous motion patterns similar to those used in
previous studies (e.g., Ledgeway, 1994; Ledgeway &
Smith, 1994b) and were again composed of LM, CM, or
OM. They were constructed from the sum of two
horizontal sinusoidal modulations (components) of the
same spatial and temporal frequency (1 Hz) drifting
smoothly in opposite directions. The orientation of the
drifting spatial modulation (horizontal) was always
orthogonal to that of the 1-D dynamic noise (vertical) in
all cases to ensure that any aftereffects of adaptation were
due to the presence of the first- or second-order test
structure and not the carrier (see Culham et al., 1998). The
spatial frequency of the sinusoidal modulation could differ
from that of the adaptation stimuli (0.5 c/deg) by j2, j1,
0, 1, or 2 octaves to measure the spatial-frequency
dependence of the dMAE. The visibilities of the test
stimuli were equated by presenting each pattern at the
same multiple of threshold (3.65 × threshold for A.J.S. and
C.V.H. and 3.1 × threshold for P.D.J.). Thus, the modu-
lation depths of the adaptation stimuli were 2.74 × higher
than those of the test stimuli for all observers. Although
equating stimuli in this manner has become conventional
practice in the literature, it does assume that the
magnitude of the internal (visual) response scales linearly
with multiples of threshold in each case. However,
presenting first- and second-order stimuli at equal multi-
ples of threshold may not be unreasonable, as it often
leads to quantitatively similar performance for the two
More intriguing information
1. Searching Threshold Inflation for India2. The name is absent
3. Informal Labour and Credit Markets: A Survey.
4. Three Strikes and You.re Out: Reply to Cooper and Willis
5. National urban policy responses in the European Union: Towards a European urban policy?
6. CONSIDERATIONS CONCERNING THE ROLE OF ACCOUNTING AS INFORMATIONAL SYSTEM AND ASSISTANCE OF DECISION
7. The Determinants of Individual Trade Policy Preferences: International Survey Evidence
8. THE EFFECT OF MARKETING COOPERATIVES ON COST-REDUCING PROCESS INNOVATION ACTIVITY
9. The name is absent
10. The Structure Performance Hypothesis and The Efficient Structure Performance Hypothesis-Revisited: The Case of Agribusiness Commodity and Food Products Truck Carriers in the South