Journal of Vision (2007) 7(8):1, 1-12
O 50
w
eŋ
a) Test LM
- CVH
• AJS
PDJ
25
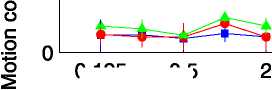
0.125 0.5
Schofield, Ledgeway, & Hutchinson
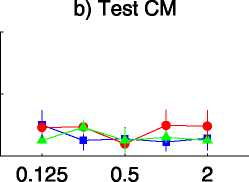
Test spatial frequency (c∕deg)
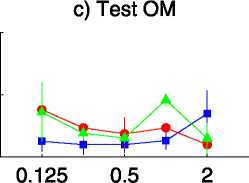
Figure 4. Sensitivity to motion contrast in test. Circles, squares, and triangles represent data for observers A.J.S., C.V.H., and P.D.J.,
respectively. Error bars are as described in Figure 1.
frequency. Adaptation to LM also induced an aftereffect
(CPSE , 40%) onto OM tests (Figure 3c, LM:OM),
although, in the case of P.D.J., this was very weak. The
effect was much less well tuned for spatial frequency than
either the LM:LM or the LM:CM interaction.
Adaptation to CM induced a moderate aftereffect
(CPSE , 50%) in CM tests (Figure 3e, CM:CM). This
effect was reasonably well tuned for spatial frequency, but
this tuning was broader than that found in the LM:LM
case. Although the effect peaked at 1 c/deg (+1 octave) for
two of three observers, the tuning was asymmetric for at
least one of these observers, suggesting that their peak
may have been poorly estimated. However, we cannot rule
out the possibility that the adaptable CM channels are
more widely spaced than those for LM and that we
adapted to one side of the center frequency of the nearest
channel for two of our three observers. Adaptation to CM
induced a moderate aftereffect (CPSE , 40%) onto OM
tests (Figure 3f, CM:OM). This effect showed some
spatial-frequency tuning. Adaptation to CM induced only
a very weak shift in PSE for LM tests (Figure 3d, CM:
LM). With the exception of one point (2 c/deg for A.J.S.),
the PSE did not exceed a motion contrast of 20%. This
very small effect was clearly not tuned for spatial
frequency.
Adaptation to OM induced a moderate aftereffect
(CPSE , 50%) onto OM tests (Figure 3i, OM:OM). The
effect was, at best, very broadly tuned and possibly low pass.
Adaptation to OM induced only very weak and broadly
tuned aftereffects (CPSE e 20%) onto CM (Figure 3h, OM:
CM) and LM (Figure 3g, OM:LM) tests.
We also assessed observer sensitivity to our test cues by
extracting just-noticeable differences (75% correct thresh-
olds) from the logistic fits for the control conditions where
observers adapted to the carrier only. These data (shown
in Figure 4) confirm that observers were quite sensitive to
changes in motion contrast.
We estimated the transfer of adaptation between cues
for each observer. The percentage transfer (T) was
calculated from the equation T = 100 (x/w), where x is
the between-cue PSE at 0.5 c/deg and w is the within-cue
PSE at 0.5 c/deg for the adapter. We used the central PSE
rather than those for all spatial frequencies as otherwise
estimated transfer was very sensitive to the relative tuning
of the aftereffects being compared. Table 1 shows the
estimated percentage transfers for each observer. These
results confirm the subjective impression gained from
inspecting Figure 3 that adaptation to an LM signal
transfers only partially to CM and OM tests, whereas
adaptation to CM transfers well to OM but only very
weakly to LM and adaptation to OM transfers only very
weakly to LM and CM.
Test (%)
Adaptation |
Observer |
LM |
CM |
OM |
LM |
C.V.H. |
44.33 |
66.77 | |
A.J.S. |
56.22 |
26.79 | ||
P.D.J. |
42.97 |
18.21 | ||
CM |
C.V.H. |
24.56 |
110.91 | |
A.J.S. |
31.34 |
64.48 | ||
P.D.J. |
29.28 |
100.61 | ||
OM |
C.V.H. |
1.44 |
27.16 | |
A.J.S. |
18.23 |
21.93 | ||
P.D.J. |
23.63 |
0 |
Table 1. Percentage transfer of adaptation between cues. See text
for details.
Discussion
We have characterized the spatial- and temporal-
frequency responses of human vision to moving OM
stimuli and compared these to sensitivities measured for
LM and CM under similar test conditions. OM sensitivity
curves are both spatially and temporally low pass, but OM
detection has reasonably high temporal acuity and, thus,
OM is unlikely to be a third-order cue as defined by Lu
and Sperling (2001). We have also tested the ability of
our motion cues to induce the dMAE onto patterns of a
similar type and assessed the degree to which the dMAE
More intriguing information
1. IMMIGRATION POLICY AND THE AGRICULTURAL LABOR MARKET: THE EFFECT ON JOB DURATION2. The purpose of this paper is to report on the 2008 inaugural Equal Opportunities Conference held at the University of East Anglia, Norwich
3. The name is absent
4. Ein pragmatisierter Kalkul des naturlichen Schlieβens nebst Metatheorie
5. The name is absent
6. Evaluating Consumer Usage of Nutritional Labeling: The Influence of Socio-Economic Characteristics
7. Informal Labour and Credit Markets: A Survey.
8. THE MEXICAN HOG INDUSTRY: MOVING BEYOND 2003
9. The name is absent
10. The name is absent