Journal of Vision (2007) 7(8):1, 1-12
Schofield, Ledgeway, & Hutchinson
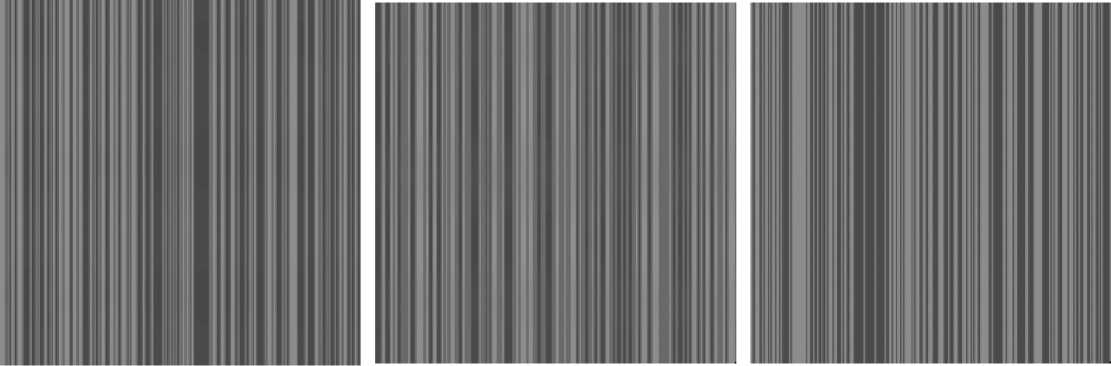
Movies 4-6. Sample test sequences for Experiment 3. Test sequences comprised two counterphasing components that are fully balanced
in these examples so as to produce “flicker” rather than motion. Left panel, Movie 4, LM; middle panel, Movie 5, CM; right panel, Movie 6,
OM. Test sequences lasted for 1 s. As static images, only the first unmodulated frame is shown. The reader is referred to Movies 1 -3,
which better illustrate our cues in a static presentation.
varieties of motion and at least goes some way toward
controlling for gross differences in sensitivity.
A nulling technique was employed to measure the
magnitude of the aftereffect. Specifically, the relative
modulation depths of the oppositely moving components
of the test stimuli could be manipulated in a “seesaw”
(antagonistic) fashion to null the perception of unidirec-
tional motion following adaptation. For example, if the
modulation depth of the upward-moving component was
increased by 10%, that of the downward component
decreased by 10% accordingly. In this manner, the overall
modulation depths of the test stimuli were kept constant.
We express the relative modulation depths of the two
motion components in terms of “motion contrast” (C),
calculated as a Michelson ratio; specifically, C = 100(Ma j
Mu)/(Ma + Mu), where Ma is the modulation depth of the
component moving in the direction of adaptation and Mu
is the modulation depth of the opposite-moving compo-
nent. Note that when the two components had equal
modulation depth, motion contrast was zero.
Procedure
At the beginning of each run of trials, the adaptation
stimulus (a sinusoidal modulation drifting either upward or
downward) was presented for 2 min followed by the
presentation of a blank field (except for the central fixation
spot) to indicate to the observer that the test stimulus was
to be presented 0.5 s later. The test stimulus was present
for a total of 1 s, and the observer’s task was to indicate its
overall perceived direction of drift (i.e., either upward or
downward). Adaptation was then “topped up” with a 10-s
presentation of the adaptation stimulus before the test
stimulus was presented again, and so on.
The motion contrast of the test stimulus was chosen at
random from a set of seven predetermined values. These
ranged from j100% (all motion was opposite adaptation)
to 100% (all motion was in the same direction as
adaptation) in equal steps of 33.3%.
Observers completed between two and six runs of
70 trials for each test spatial frequency, and all possible
combinations of the first- and second-order adaptation and
test stimuli were examined to investigate within-cue and
between-cue adaptation effects. Adaptation cues were
presented moving either up or down, in separate runs,
with an equal number of runs per direction. We also
measured the effects of adapting to the carrier only on each
test cue. Observers were required to take a minimum break
of 2 hr between runs with different adaptation stimuli to
minimize any carryover of adaptation between conditions.
Analysis
Data were collapsed across direction of motion in the
adaptation phase, and logistic functions were fitted to the
resulting data for each observer and condition, plotted as
the percentage of trials on which the observer perceived
the test stimulus to drift in the same direction as
adaptation as a function of the motion contrast of the test.
From these fits, we determined the motion contrast, which
produced no net perceived direction of drift (i.e., the point
of subjective equality [PSE]).
Results
Figure 3 shows the PSEs for each observer and all
combinations of adapter and test cue. Results for LM,
More intriguing information
1. Education and Development: The Issues and the Evidence2. Should informal sector be subsidised?
3. An Intertemporal Benchmark Model for Turkey’s Current Account
4. Technological progress, organizational change and the size of the Human Resources Department
5. Gender and aquaculture: sharing the benefits equitably
6. Clinical Teaching and OSCE in Pediatrics
7. Globalization, Divergence and Stagnation
8. Concerns for Equity and the Optimal Co-Payments for Publicly Provided Health Care
9. The name is absent
10. The name is absent