= 2
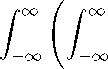
1 {|M_a|<gn}CT4(u)Lχ(r, u)du
f∞∞ 1 {∣u-a∣<ξn}LX (1 ,u )d u
''
Lχ(r, a)da + θa.s.(1)
= 2
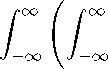
a.s. 2
1 {∣zξn∣<ξn}σ4( a + zξn ) LX ( r, a + zξn )d z
f∞∞ 1 {∣zξn∣<ξn}LX(1, a + zξn)dz
Lχ(r, a)da + oa,s. (1)
Proof of Step 4:
Z∞
-∞
Lχ(r, a)2
MM d a.
(20)
Cn,r
1 L (n_ 1) rJ
l σ 2( Xs )d s
0
√= £ σ2(Xi/n) --n
V n
i =1
1 l ( n_ 1)rJ l ( n_ 1)rJ ∕∙( i+1) /n
-= £ σ2(Xi/n) -√n £ I σ2(Xs)ds
nn i =1 i =1 7i/n
l ( n_ 1)rJ r( i+1) /n
n £
i=1 i/n
(σ 2( Xi/n )
- σ2(Xs)) ds
(21)
and, given the Lipschitz assumption on σ2(∙), the last line in (21) is oP(1) by the same
argument as the one used in Step 1.
Given Steps 1-4 above, it follows that the quadratic variation process of Zn,r is given by
2 [∞ σ4 (a) Lx(r, a)da + 2 [∞ σ4 (a) Lχ(^ a).2 da - 4 [∞ σ4 (a) Lχ(^ a).2 da
-∞ -∞ LX (1, a) -
∞ LX (1, a)
= 2 [∞ σ4 (a) Lχ(r, a)(LX(1,a) - Lχ(r, a)) da. (22)
∞ -QQ LX (1 ,a)
The statement in the theorem then follows.
(i)b Without loss of generality, suppose that r < r'. By noting that
1 l ( n_ 1)rJ l ( n_ 1)rJ
-= £ sn (Xi/n ) -√n £ (Xi+1 /n - Xi/n )2
nn i=1 i =1
1 [( n_ 1) r' ] [( n_ 1) r' ]
= — £ sn(Xi/n) -Vn £ (Xi+1 /n - Xi/n)2,
n i=1 i=1
with Sn(Xi/n) = 0 and (Xi+1 /,n - Xi/n)2 = 0 for i > L(n - 1)rJ, the result then follows by
the continuous mapping theorem.
22
More intriguing information
1. Consciousness, cognition, and the hierarchy of context: extending the global neuronal workspace model2. CONSUMER ACCEPTANCE OF GENETICALLY MODIFIED FOODS
3. If our brains were simple, we would be too simple to understand them.
4. The name is absent
5. Migrant Business Networks and FDI
6. The name is absent
7. Database Search Strategies for Proteomic Data Sets Generated by Electron Capture Dissociation Mass Spectrometry
8. The name is absent
9. On Social and Market Sanctions in Deterring non Compliance in Pollution Standards
10. THE USE OF EXTRANEOUS INFORMATION IN THE DEVELOPMENT OF A POLICY SIMULATION MODEL